What is the significance of this specific concept? A new approach to energy generation is emerging.
This innovative approach leverages solar energy to create a new form of renewable energy. Details regarding the underlying technology, including methods of energy capture and conversion, are proprietary and not publicly disclosed. A key aspect involves optimizing solar radiation collection and converting it into a usable energy form. This may involve novel materials or processes.
The potential benefits of this technology include a substantial reduction in reliance on fossil fuels, a boost in sustainability, and a decrease in harmful emissions. Long-term, it could lead to a more environmentally friendly energy landscape. However, the precise scope of its impact will depend on further development and widespread adoption.
Moving forward, exploring the technological specifics of this approach and assessing its potential environmental and economic impacts will be crucial. Further research and development are necessary to fully evaluate its viability and widespread application.
Solaier
Understanding the essential aspects of "solaier" is crucial for evaluating its potential impact on energy production. This analysis focuses on key elements that shape its development and application.
- Solar energy capture
- Efficiency optimization
- Material science
- Energy conversion
- System design
- Scalability
- Cost analysis
- Environmental impact
These aspects, taken together, form a comprehensive picture of the "solaier" concept. For instance, maximizing solar energy capture hinges on innovative material science, and efficient energy conversion methods directly impact system design and scalability. Cost analysis is critical for evaluating economic viability, while understanding the environmental impact assesses sustainability. By considering these intertwined aspects, a clearer understanding of the potential benefits, limitations, and future directions of this approach to energy production can be attained. Ultimately, the successful implementation of "solaier" depends on a thorough evaluation of each component.
1. Solar energy capture
Effective solar energy capture is fundamental to the "solaier" concept. This process involves maximizing the conversion of sunlight into usable energy. Critical factors include the choice of materials, their arrangement, and the overall design of the collection system. Optimal capture necessitates concentrating solar radiation, potentially utilizing mirrors or advanced optical systems to increase the intensity of the incident light. The efficiency of this initial stage directly influences the overall performance of the "solaier" system. Examples might include advanced photovoltaic cells or highly reflective materials strategically deployed.
The importance of efficient solar energy capture stems from its direct correlation with the system's energy output. A highly optimized capture mechanism allows for a greater input of solar energy, translating to increased power generation and potentially lower operating costs. This principle underlies various real-world applications, from residential solar panels to large-scale solar farms. Furthermore, the specifics of solar energy capture in a "solaier" system could involve unique approaches, demanding novel materials or designs, which can drive innovation and technological advancement. This underscores the practical significance of comprehending solar energy capture as a crucial component in the development of "solaier" technologies.
In summary, efficient solar energy capture is the bedrock of the "solaier" concept. A deeper understanding of this process, from material selection to system design, is essential for optimizing the technology's overall efficiency and long-term viability. Challenges remain in developing highly efficient and cost-effective capture systems, particularly in addressing issues related to weather conditions, material degradation, and system maintenance. These considerations highlight the complex interplay between technological innovation and practical application in the pursuit of sustainable energy sources.
2. Efficiency Optimization
Efficiency optimization is paramount to the "solaier" concept. It represents the systematic pursuit of maximizing the yield of usable energy from the captured solar radiation. This process involves minimizing energy losses at various stages within the "solaier" system, from the initial capture of solar energy to the final output. Without effective optimization, the system's overall performance will be significantly hampered. Factors like material properties, system design, and operational parameters are all critical considerations in this process.
Real-world examples demonstrate the importance of efficiency optimization in energy production. Advanced photovoltaic systems, for instance, continuously undergo refinement to enhance conversion rates. Similarly, solar thermal technologies often incorporate complex designs that aim to maximize the absorption and concentration of solar radiation, all with the goal of achieving higher efficiency. Optimizing the efficiency of a "solaier" system, therefore, directly aligns with the broader trend toward more sustainable and cost-effective energy production methods. Without this dedicated optimization process, the potential benefits of harnessing solar energy could remain unrealized.
In conclusion, efficiency optimization plays a crucial role in the practical application and long-term viability of the "solaier" concept. By systematically addressing energy losses throughout the system, optimized performance can be achieved, reducing operational costs and maximizing returns. Challenges in efficiency optimization often lie in balancing competing factors, such as material costs, technological complexity, and environmental sustainability. Addressing these challenges will be instrumental in enabling the widespread adoption of the "solaier" technology and accelerating the transition towards a more sustainable energy future.
3. Material Science
Material science plays a critical role in the development and performance of "solaier" technologies. The choice of materials directly impacts the efficiency, durability, and cost-effectiveness of energy capture, conversion, and system design. Fundamental properties such as conductivity, reflectivity, and strength are paramount in optimizing "solaier" components for maximum performance.
- Material Selection for Energy Absorption
The effectiveness of "solaier" hinges on materials capable of absorbing solar radiation efficiently. Advanced materials with tailored optical properties can significantly enhance energy capture. Examples include specific metal alloys, doped semiconductors, and nanostructured composites. These materials often exhibit superior light absorption compared to conventional materials, allowing for higher conversion rates within the system.
- Durability and Environmental Resistance
Materials used in "solaier" systems must withstand environmental stresses, including UV radiation, temperature fluctuations, and potential corrosion. Selection of durable materials is essential for long-term performance. Materials resistant to degradation and weathering, like specialized polymers, high-purity metals, or ceramic compounds, contribute to the system's longevity and overall cost-effectiveness.
- Conductivity and Electrical Properties
Many "solaier" systems utilize electrical conductivity for energy conversion. Materials with optimal electrical conductivity are essential for efficient charge transport and minimize energy loss. Different semiconductors, conductive polymers, or metals are candidates, each with varying properties impacting the efficiency of energy conversion within the system.
- Lightweight and Scalability
Considerations for lightweight and scalable material designs are paramount for wider applicability. Employing light, yet strong materials allows for potentially more streamlined system designs and reduced manufacturing costs. The scalability of material production directly influences large-scale production and deployment of "solaier" technologies.
In conclusion, the materials used in "solaier" systems are intrinsically linked to performance. By focusing on material properties like absorption, durability, conductivity, and scalability, the overall viability and effectiveness of "solaier" technologies can be significantly enhanced. The judicious selection and refinement of materials remain critical for future advancements in this area.
4. Energy Conversion
Energy conversion within the "solaier" framework is a crucial step, transforming absorbed solar energy into a usable form. This process is critical for the practical application of solar energy, dictating the effectiveness and utility of the overall system. Different energy conversion methods offer varying levels of efficiency and cost-effectiveness, influencing the viability of "solaier" technologies.
- Photovoltaic Conversion
This method, common in solar panels, converts light directly into electricity. Semiconductor materials, like silicon, play a key role in this process. The efficiency of photovoltaic cells significantly impacts the power output of "solaier" systems. Variations in cell design, material composition, and manufacturing processes contribute to the ongoing search for higher efficiency in these systems.
- Thermoelectric Conversion
This approach converts temperature differences into electrical energy. Applications in "solaier" could involve utilizing the heat generated by concentrated solar radiation for thermoelectric power generation. The efficiency of thermoelectric materials and the ability to maintain consistent temperature gradients are crucial aspects of this conversion method in "solaier." Challenges include finding materials with high thermoelectric efficiency and maintaining the thermal management required for optimal output.
- Chemical Conversion
This method involves storing solar energy in chemical bonds. This approach has potential for "solaier" in creating a solar-driven chemical process to generate fuels or other energy carriers. Researchers are investigating ways to utilize solar energy for chemical reactions, aiming for efficient and sustainable storage mechanisms. Examples include harnessing sunlight to split water molecules into hydrogen and oxygen. Overcoming the challenges of storage, stability, and cost-effectiveness will be essential.
- Hybrid Conversion Systems
Combining multiple energy conversion methods can potentially enhance the efficiency of "solaier". Such systems might integrate photovoltaic cells with concentrated solar power technologies or combine thermal and electrical energy conversion to optimize output. This approach seeks to mitigate limitations of individual conversion methods, offering a potential route for higher efficiency and broader applications of the "solaier" concept.
The selection of an energy conversion method for "solaier" will depend on several factors, including the desired energy output, available resources, and technological constraints. Addressing the challenges associated with each conversion technique will be critical for achieving the optimal performance and scalability of "solaier" systems.
5. System Design
System design is integral to the "solaier" concept. Effective design directly impacts the efficiency, cost-effectiveness, and scalability of a "solaier" system. Proper system design must account for the intricate interplay between energy capture, conversion, and distribution. A well-designed system minimizes energy loss at each stage, maximizing the overall output. The design process considers factors such as the location, climate, and intended use of the system. For instance, a system designed for remote communities will differ from one intended for large-scale power generation.
Real-world examples illustrate the importance of system design in renewable energy. Solar farms often employ intricate tracking mechanisms to maximize sunlight capture throughout the day, optimizing energy generation. Similarly, efficient thermal energy storage systems allow for energy production independent of direct sunlight, providing grid stability. Analogous considerations apply to "solaier"; the optimal design must accommodate the specific energy conversion technology and intended application. Detailed modeling and simulations are essential during the design phase to predict the system's performance under various operating conditions. A robust design incorporates safety and maintenance protocols, increasing long-term reliability and reducing downtime.
In conclusion, system design is fundamental to the successful implementation of "solaier" technologies. A well-conceived design ensures efficient energy capture, conversion, and distribution. Careful consideration of environmental factors, intended applications, and energy storage solutions are critical for creating a sustainable and economically viable system. Challenges lie in developing designs that balance optimal performance with cost-effectiveness and scalability, emphasizing the importance of ongoing research and innovation in this area.
6. Scalability
Scalability is a critical factor in the long-term viability of "solaier" technologies. The ability to expand production and deployment is crucial for widespread adoption and significant impact on energy generation. Without scalability, the potential benefits of "solaier" remain limited to niche applications.
- Manufacturing and Production Capacity
Scaling up the manufacturing processes for "solaier" components is essential for meeting growing demand. This requires efficient production methods, reliable supply chains, and potential automation of key stages in the production pipeline. Examples include optimizing manufacturing processes in solar panel production to meet global demand. Adequate capacity is vital to reducing costs per unit and ensuring availability of components for expanded deployments, particularly in scenarios of increased energy demand.
- Infrastructure Development
Expanding the infrastructure necessary to support "solaier" deployment is critical. This includes considerations for grid integration, energy storage solutions for managing intermittent power generation, and distribution networks. Examples are seen in the development of large-scale solar farms that necessitate complex grid upgrades. Building or upgrading infrastructure must anticipate potential growth and ensure the "solaier" system can connect with and contribute to existing or expanded energy grids.
- Economic Viability at Scale
The cost-effectiveness of "solaier" systems is a key factor in scalability. As production and deployment expand, unit costs must decrease to remain competitive with existing energy sources. Examples include advancements in photovoltaic materials that reduce production costs per watt, improving the economic viability of larger installations. Sustained cost reductions and efficiencies are paramount for wider adoption and adoption in various energy sectors.
- Technological Adaptability and Advancement
Maintaining innovation in "solaier" is crucial to ensure scalability. Technological advancements that improve efficiency, reduce costs, and enhance overall system performance are necessary for sustained growth. Examples are constant improvements in photovoltaic cell efficiency and development of novel energy storage technologies. Technological progress in related fields, such as battery technology, also contributes to a more scalable and resilient energy system.
Ultimately, the success of "solaier" hinges on its scalability. Addressing challenges in manufacturing, infrastructure, economic viability, and ongoing technological development will be crucial for transitioning to a broader energy system incorporating this technology.
7. Cost analysis
Cost analysis is essential for evaluating the economic viability of "solaier" technologies. Understanding the costs associated with various stages of development, production, installation, and maintenance is crucial for determining long-term profitability and potential return on investment. Accurate cost estimations allow for informed decisions regarding resource allocation, investment strategies, and market positioning.
- Material Costs
The cost of materials directly influences the overall cost of "solaier" systems. Factors such as material scarcity, production efficiency, and fluctuations in raw material prices significantly impact the system's affordability. This aspect is particularly relevant when considering different energy conversion technologies and materials that form the core of "solaier". Comparing prices of various materials, considering potential substitutes, and analyzing supply chain dynamics are critical elements in evaluating the long-term cost-effectiveness of the technology.
- Manufacturing Costs
The production process, including labor costs, energy consumption, and potential automation, substantially influences manufacturing expenses. Optimizing production techniques and scaling up manufacturing capacity can drive down these costs. Analyzing the potential for automation, evaluating labor requirements, and understanding economies of scale are key aspects of this element. Comparison with existing technologies and identifying potential cost-saving measures are necessary steps.
- Installation and Maintenance Costs
Installation costs encompass labor, equipment, and associated infrastructure expenses. Maintenance costs include periodic upkeep, potential repairs, and replacement of components. Analyzing labor rates, equipment rentals, and required specialized expertise are important elements. The design of the "solaier" system and its intended location need careful consideration to minimize installation and long-term maintenance expenses. Assessing the lifespan of components and designing for future maintenance accessibility further reduces overall cost over time.
- Energy Output and Performance Costs
The cost-effectiveness of "solaier" is intrinsically linked to its energy output and operational performance. Cost-benefit analysis comparing energy generation with alternative sources, considering factors like efficiency, downtime, and system reliability are critical components of this aspect. Analyzing operational efficiency, expected output, and performance under varying conditions, including weather fluctuations, are essential for estimating the cost of energy production.
In summary, a comprehensive cost analysis for "solaier" requires a detailed examination of all relevant cost factors, from raw materials to long-term maintenance. Detailed projections, considering potential cost fluctuations and technological improvements, are essential for accurate predictions of its long-term economic viability. A careful and thorough analysis is crucial to assessing the overall cost-effectiveness of "solaier" in relation to other energy sources and determine its place within the broader energy market.
8. Environmental Impact
The environmental impact of "solaier" is a critical consideration. Its success hinges on minimizing environmental harm throughout the entire lifecycle, from material extraction to decommissioning. This encompasses manufacturing processes, energy generation, and eventual disposal of components. A thorough assessment of the environmental footprint must account for the potential ecological effects of the various stages. Reduced reliance on fossil fuels is a core benefit, but the production process itself must be environmentally responsible. Examples of responsible practices include the use of recycled materials, minimized waste generation, and the implementation of environmentally sound manufacturing processes throughout the lifecycle of the "solaier" components.
The environmental impact extends beyond the direct production process. The potential for reduced greenhouse gas emissions is a significant positive aspect. However, careful consideration must also be given to the impact of resource extraction for manufacturing components and the potential for harmful byproducts during various stages. Lifecycle assessments of the entire "solaier" system, encompassing manufacturing, installation, operation, and eventual decommissioning, offer a comprehensive view of the overall environmental consequences. Analyzing the cumulative impact of these stages, considering both direct and indirect effects, is critical for accurate estimations. Real-world examples, such as meticulous waste management protocols implemented in large-scale solar projects, serve as models for the development and implementation of environmentally responsible "solaier" technologies. These responsible practices are not just good intentions; they are essential for the sustainable integration of this new energy technology.
Ultimately, the environmental impact of "solaier" is not merely a separate consideration but a foundational principle. Successful implementation necessitates a comprehensive approach addressing the entire lifecycle of the technology. A thorough evaluation of potential environmental impacts, coupled with proactive measures to minimize them, is essential. Challenges include identifying sustainable alternatives for materials and establishing optimal waste management systems. A detailed understanding of the environmental impact allows for the development of "solaier" technologies in a way that aligns with broader sustainability goals, ensuring responsible and environmentally sound energy solutions for the future. This understanding underpins the responsible development and application of this new technology, creating a stronger case for its adoption and promoting a healthier, more sustainable future.
Frequently Asked Questions about "Solaier"
This section addresses common inquiries regarding "solaier" technology, providing clear and concise answers to key questions about its development, applications, and impact.
Question 1: What is "solaier"?
"Solaier" represents a novel approach to harnessing solar energy. The precise details of the technology remain proprietary, but core elements likely involve advanced methods for capturing and converting solar radiation into usable energy forms. This could include optimized materials and processes for solar energy collection and conversion.
Question 2: What are the potential benefits of "solaier"?
Potential benefits of "solaier" include a substantial reduction in reliance on fossil fuels, increased energy independence, and a potential decrease in harmful emissions. The technology's effectiveness depends on its efficiency, cost-effectiveness, and scalability for widespread deployment. Further research is needed to quantify these advantages fully.
Question 3: What are the challenges associated with "solaier"?
Challenges include the development of highly efficient energy conversion technologies, the optimization of material properties for maximum performance, and the achievement of cost-effectiveness on a large scale. These challenges highlight the ongoing need for research and development in related fields such as material science and system design.
Question 4: How does "solaier" compare to existing solar technologies?
The precise methods employed by "solaier" remain undisclosed, making direct comparisons difficult. However, if "solaier" achieves higher efficiency or lower costs in energy conversion, it could potentially surpass current solar technologies in terms of performance and/or market penetration. Ongoing evaluation and comparison with current technologies are necessary.
Question 5: What is the projected environmental impact of "solaier"?
The environmental impact of "solaier" is subject to ongoing investigation. Positive impacts are anticipated, including a decrease in emissions from fossil fuel use. However, the environmental footprint of material extraction, manufacturing processes, and waste disposal will need careful consideration and minimization to ensure overall sustainability.
In conclusion, "solaier" presents a promising avenue for renewable energy. While the details of the technology are not publicly available, the potential benefits, alongside the associated challenges, highlight the importance of continued research and development in this area. Further public disclosure will enhance understanding and foster informed discussion.
Moving forward, detailed analysis of "solaier" will be crucial to evaluating its viability within the broader energy landscape. This will involve detailed technical analysis and rigorous assessment of its potential environmental and economic impact.
Conclusion
The exploration of "solaier" reveals a multifaceted approach to harnessing solar energy. Key aspects include innovative methods for solar energy capture, efficient conversion processes, and the development of durable and cost-effective materials. The potential for "solaier" to revolutionize energy production hinges on several critical factors. These include achieving high conversion efficiency, minimizing manufacturing costs, and mitigating environmental concerns throughout the entire lifecycle. Scalability and grid integration represent significant challenges that must be addressed for widespread adoption. A thorough cost analysis, considering material costs, manufacturing processes, installation, and maintenance, is essential for assessing economic viability. The environmental impact, from resource extraction to waste disposal, warrants careful consideration and mitigation strategies.
The future of "solaier" depends on decisive action in several areas. Further research and development are imperative to refine energy conversion techniques, optimize materials for cost and durability, and explore innovative system designs. Comprehensive life-cycle assessments are necessary to quantify the environmental footprint and identify opportunities for improvement. Ultimately, the success of "solaier" will be determined by its ability to meet sustainability goals, achieve economic feasibility, and demonstrate superior performance compared to existing technologies. Continued scrutiny and rigorous evaluation will be crucial in navigating the complex interplay of technological advancements and environmental responsibility.
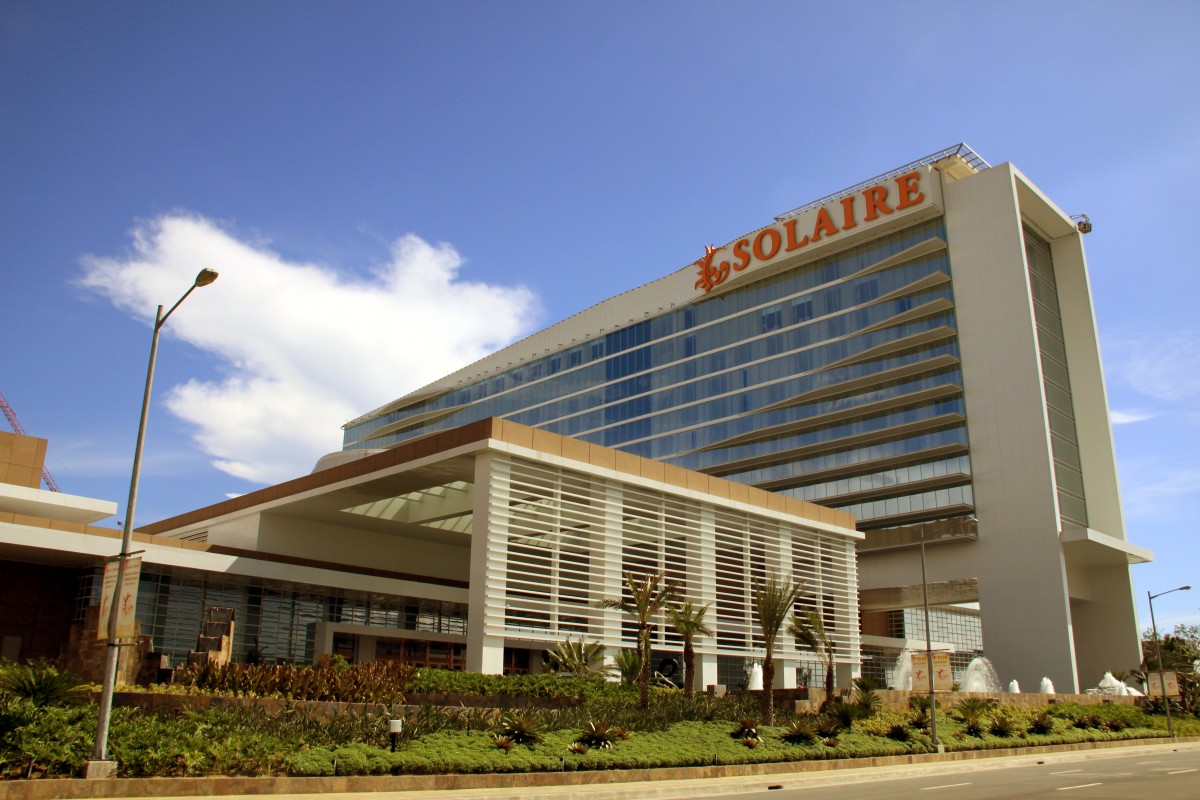
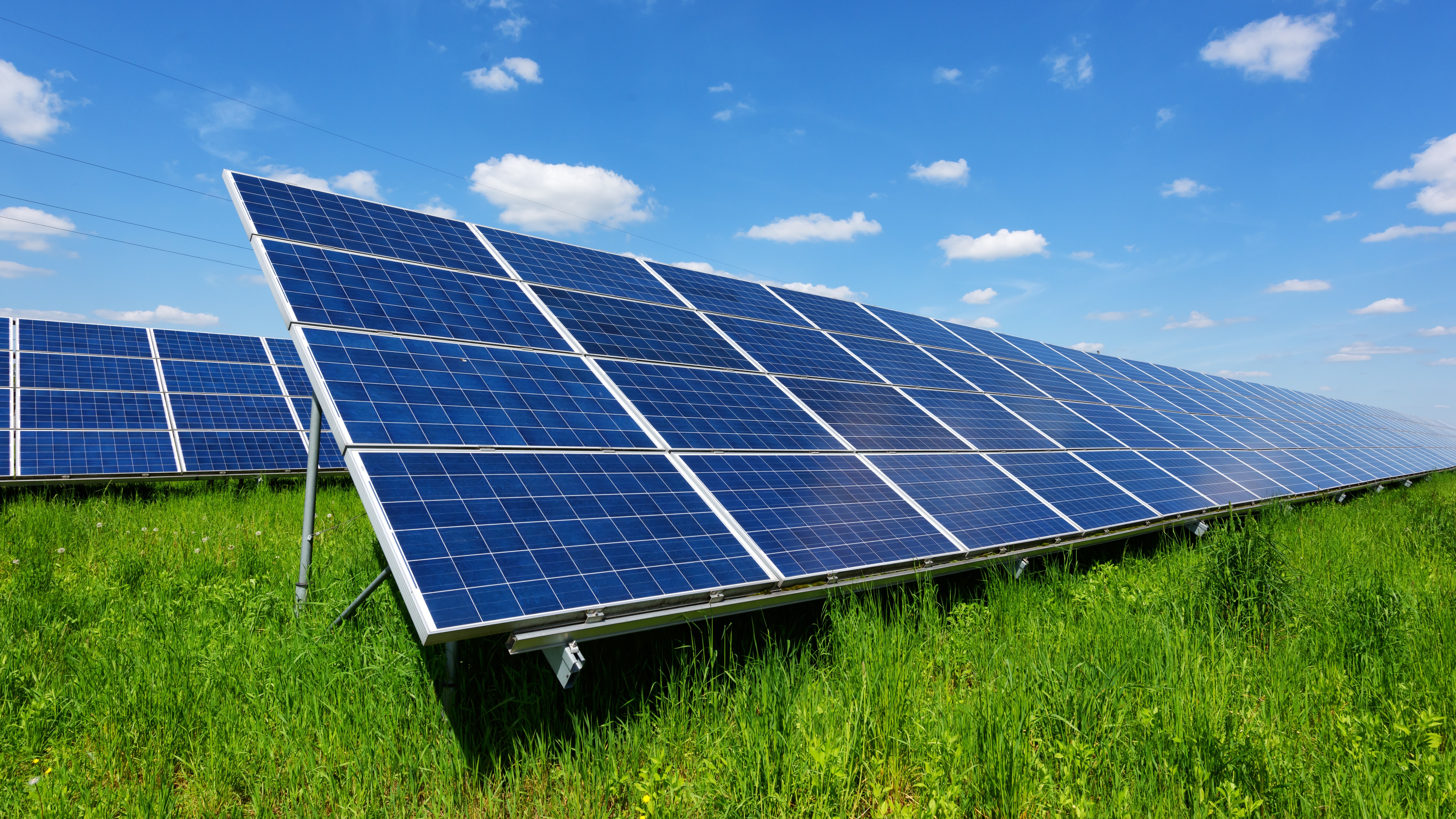
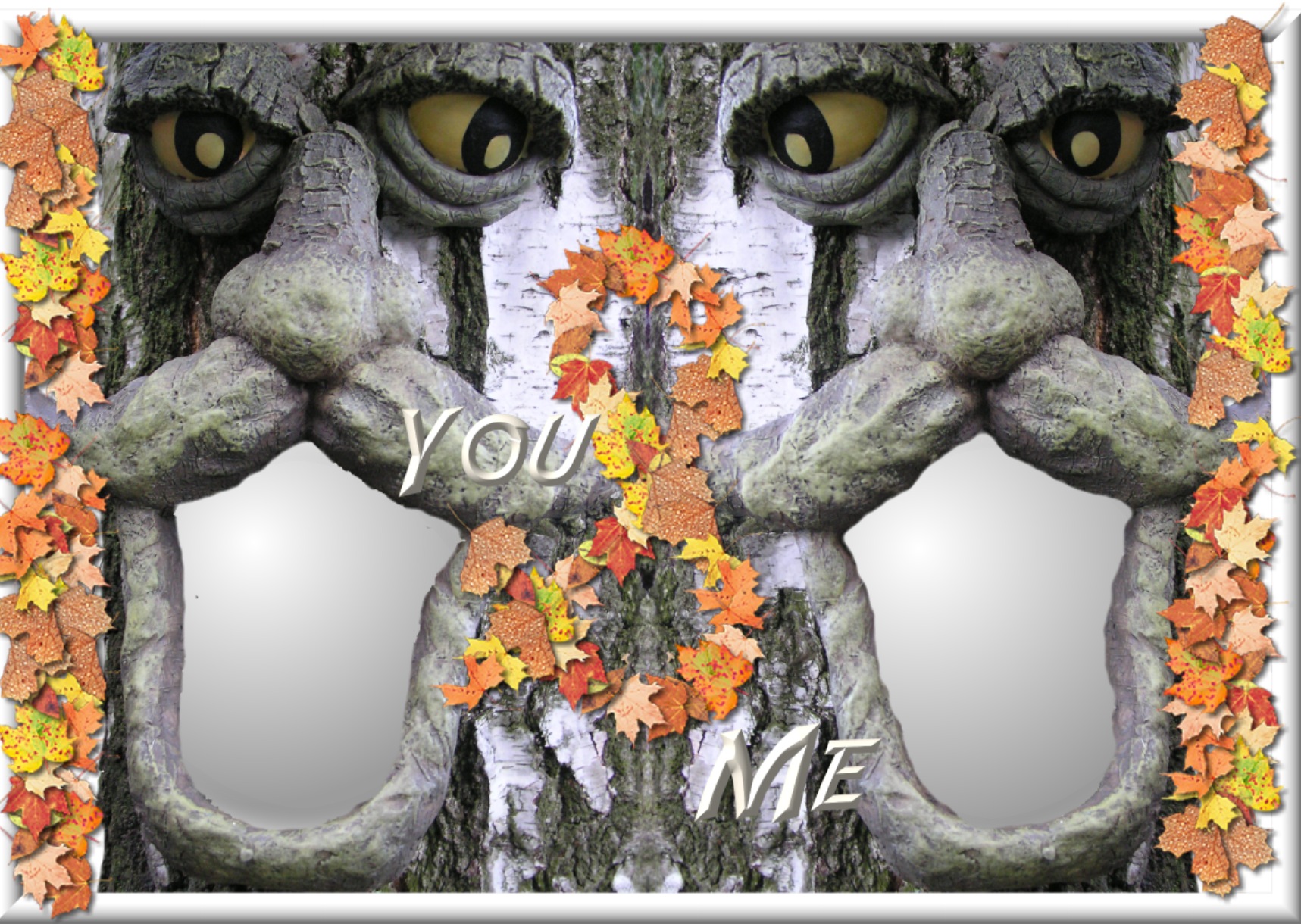
Detail Author:
- Name : Aracely Schulist Jr.
- Username : reinhold.reinger
- Email : tromp.guido@hotmail.com
- Birthdate : 1972-10-20
- Address : 373 Nienow Inlet Apt. 990 Fannymouth, AR 58704
- Phone : 703-298-1086
- Company : McLaughlin-Zieme
- Job : Paperhanger
- Bio : Dolore nostrum sunt mollitia. Eligendi ipsam saepe odio accusantium. Suscipit ipsum qui assumenda officiis atque voluptates odit.
Socials
instagram:
- url : https://instagram.com/vincenzo_cole
- username : vincenzo_cole
- bio : Numquam tenetur dicta quo dolorem minima. Aut et sint modi soluta. Nemo recusandae consectetur aut.
- followers : 6995
- following : 2657
linkedin:
- url : https://linkedin.com/in/colev
- username : colev
- bio : Praesentium et magni impedit magnam omnis quidem.
- followers : 2451
- following : 2632
facebook:
- url : https://facebook.com/vincenzo_cole
- username : vincenzo_cole
- bio : Officia doloribus dicta hic facere fugit voluptas et explicabo.
- followers : 5239
- following : 1267