What is the significance of a measure of change in photon properties? A quantifiable shift in photon characteristics holds immense potential.
A change in the properties of a photon, be it its energy, momentum, or polarization, can be precisely quantified. This measurement, representing a shift in these fundamental light particle attributes, provides a means to understand and potentially control light-matter interactions. For instance, a shift in a photon's energy might correlate with specific material interactions, providing valuable insights into the material's properties. This precise measurement of variation in photon characteristics has wide-ranging implications in various fields.
This quantifiable variation in photon properties is crucial in numerous applications. Its impact extends to areas such as quantum computing, where precise control over photon states is essential. Further, it aids in the development of advanced imaging techniques with enhanced resolution and sensitivity. The study and understanding of this variation also holds promise for advancing our understanding of fundamental physical processes.
This discussion will now proceed to examine the various applications of this measurable shift in photon characteristics, addressing its roles in quantum optics, material science, and medical imaging.
Photon Variation
Precise measurement of photon changes is critical for advancing various fields, from quantum computing to material science. This quantifiable shift, or photondelta, provides insights into the fundamental behavior of light and matter interactions.
- Energy shift
- Momentum change
- Polarization alteration
- Quantum entanglement
- Material interaction
- Imaging enhancement
- Quantum computing
- Light-matter interaction
These aspects collectively define photondelta's multifaceted nature. Energy shifts, for example, can reveal how light interacts with matter at the atomic level. Momentum changes impact the propagation of photons, crucial for designing optical devices. Understanding polarization alterations is vital for quantum communication. The ability to observe and control photon variations is critical for harnessing quantum entanglement and enabling complex quantum computing. Examining material interactions through photondelta yields valuable information on material properties. Advanced imaging techniques benefit from the precise control and measurement of these changes, enhancing resolution. Ultimately, photondelta lies at the heart of our understanding of light and its multifaceted interplay with matter, shaping future technological advancements.
1. Energy Shift
Energy shift represents a change in the energy of a photon. This change is a fundamental component of photondelta, the quantifiable variation in photon properties. A photon's energy is intrinsically linked to its frequency and wavelength. Any alteration in these properties directly corresponds to a change in energy. This energy shift is crucial for understanding how light interacts with matter. For example, when light interacts with an atom, the photon might lose energy, transitioning to a lower energy level. Conversely, a photon might gain energy when interacting with an excited atom.
The practical significance of understanding energy shift within the context of photondelta is profound. In spectroscopy, the energy shifts observed when light interacts with specific materials provide insights into the atomic and molecular structure of those materials. These shifts manifest as unique patterns in spectral lines, enabling identification and characterization. In quantum computing, precisely controlling energy shifts is paramount for manipulating qubits, the fundamental units of quantum information. Similarly, in advanced microscopy techniques, subtle energy shifts offer unique contrast mechanisms for enhanced image resolution and deeper understanding of samples at a nanoscale level. Photovoltaic devices rely on energy shifts to convert light into electricity. The ability to measure and control these shifts directly impacts the efficiency and design of these devices.
In summary, energy shift is a pivotal component of photondelta. The precise measurement of energy variations enables a deeper understanding of light-matter interactions. These interactions underpin numerous technological applications, from spectroscopic analysis to quantum computing and energy production. However, challenges remain in accurately measuring minute energy shifts under complex conditions. Continued research and development in this area are crucial for further advancements across diverse scientific and technological disciplines.
2. Momentum Change
Momentum change is an integral component of photondelta, signifying a variation in the momentum of a photon. A photon's momentum, a vector quantity, is directly related to its energy and wavelength. Changes in momentum, therefore, correlate with alterations in these fundamental properties. This connection is crucial for understanding light-matter interactions at a fundamental level. For example, the momentum transfer of a photon during scattering from a particle directly affects the particle's trajectory and energy. Similarly, in processes like Compton scattering, the change in a photon's momentum is precisely measured to determine the particle's characteristics.
The practical significance of comprehending momentum change within the context of photondelta extends to various applications. In optical devices, manipulating a photon's momentum allows for precise control over its direction and trajectory, enabling the design of advanced optical components. For instance, momentum changes enable the construction of highly efficient optical filters, which precisely select specific wavelengths of light. Furthermore, understanding momentum transfer is crucial for advanced imaging techniques. The momentum change experienced by a photon interacting with a sample can provide invaluable information about the sample's structure and composition. This is especially relevant in medical imaging, where momentum shifts can be used to create highly detailed images of biological tissues. Finally, the principle of momentum conservation, central to understanding momentum change, plays a vital role in designing and optimizing numerous technological applications involving light and matter interaction.
In conclusion, momentum change is a critical aspect of photondelta. Its connection to photon energy and wavelength, combined with its importance in light-matter interactions, has profound implications for various technologies. However, precise measurement of momentum changes can be challenging, particularly in complex environments. Future research needs to address these challenges and explore new applications of momentum change within the framework of photondelta.
3. Polarization Alteration
Polarization alteration, a crucial facet of photondelta, represents a change in the orientation of a photon's electric field vector. This directional shift in the oscillating electromagnetic wave constitutes a measurable variation in the photon's properties. Understanding polarization alteration provides insights into light-matter interactions, enabling the development of advanced optical technologies. Its relevance to photondelta lies in the precise quantification of these changes, revealing how materials interact with light on a fundamental level.
- Linear Polarization
Linear polarization describes a photon's electric field oscillating along a straight line. Variations in this linear polarization, such as a rotation of the oscillation axis, are a direct manifestation of photondelta. Analyzing these variations reveals details about the material through which the light has passed, for instance, the presence of birefringent crystals. This is employed in optical components for manipulating and filtering light waves, a key principle in advanced optical systems.
- Circular Polarization
Circular polarization involves the electric field vector rotating in a circle, generating a helical wave propagation pattern. Alterations in this rotational behavior, observable as a change in the handedness of the circular polarization, are indicative of photondelta. This characteristic is crucial in optical communications, allowing for the encoding of information within the rotating electric field vector. Precise control over these alterations is paramount for the development of highly efficient and secure communication channels.
- Elliptical Polarization
Elliptical polarization describes a more complex oscillation pattern, with the electric field vector tracing an ellipse. Variations in this elliptical polarization, involving changes in the eccentricity and orientation of the ellipse, are part of the measurable range of photondelta. Such variations provide detailed information about the interactions of the light with specific materials, potentially revealing subtle features of their atomic or molecular structure. These detailed observations are essential in advanced spectroscopic techniques.
- Applications in Optical Components
The measurable changes in polarization are crucial for numerous optical components. Polarization filters, used in photography and other applications, alter the polarization of light passing through. Polarization modulation techniques are used to transmit data in optical communication. The alteration and manipulation of polarization is fundamental in many optical components, and measuring these changes (photondelta) allows for greater precision and control.
In summary, polarization alteration, as a component of photondelta, underscores the importance of precise measurement in studying light-matter interactions. The diverse applications highlight the potential of manipulating polarization to advance fields ranging from communications to spectroscopy. Further advancements in measuring these alterations will continue to drive progress in various technologies.
4. Quantum Entanglement
Quantum entanglement, a phenomenon where two or more particles become interconnected, possesses a profound connection with photondelta. The entangled state of these particles exhibits correlations that extend beyond classical physics. Understanding these correlations, quantifiable as photondelta in the context of photons, offers significant implications for quantum information processing and fundamental physics. The intricate relationship between these intertwined states and the measurable changes in photon properties forms the basis for many ongoing investigations.
- Instantaneous Correlation
Entangled particles exhibit instantaneous correlations, regardless of the distance separating them. This non-local correlation is a key feature of entanglement. When a measurement is made on one entangled photon, instantaneously the corresponding property of the entangled photon is determined, even if they are light-years apart. This instantaneous correlation, a central aspect of photondelta, can be quantified and harnessed in various quantum technologies. Measuring these changes, or photondelta, in an entangled state is crucial to understanding their underlying mechanism.
- Superposition and Measurement
Entangled photons often exist in a superposition of states until a measurement is made. This inherent superposition plays a vital role in photondelta. The act of measuring one entangled photon influences the other, creating a predictable yet fundamentally quantum relationship. The resulting changes (photondelta) in the measured properties of each particle are intrinsically linked and provide insights into the nature of quantum reality and its implications for various technologies. Quantifying these changes, crucial to understanding photondelta in the context of entanglement, remains an active area of research.
- Quantum Computing Potential
Quantum entanglement is a cornerstone of quantum computing. Entangled photons can act as qubits, the fundamental units of quantum information. The quantifiable shifts in their properties, or photondelta, enable manipulation and processing of quantum information. Developing techniques for creating and maintaining entangled states of photons, and precisely measuring the changes in their properties (photondelta), is crucial to harnessing this potential. This is an essential aspect of understanding entanglement's role in advancing future quantum technologies.
- Testing Fundamental Physics
Entanglement experiments serve as rigorous tests of quantum mechanics. The correlations observed in entangled particles are highly sensitive to theoretical frameworks, which can help advance quantum mechanics. The resulting measurable changes (photondelta) in the properties of entangled photons can expose subtle discrepancies between theoretical predictions and experimental outcomes. Studying and understanding these subtle variations in measurable properties (photondelta) is pivotal in refining our understanding of quantum mechanics and its implications. This area of research is vital for developing more sophisticated quantum theories.
In conclusion, quantum entanglement's profound connection with photondelta is multifaceted. The instantaneous correlations, superposition effects, and potential for quantum computing rely on the precise measurement of changes (photondelta) in entangled photon properties. Moreover, entanglement experiments provide crucial testing grounds for fundamental physics. Further exploration and understanding of these interrelationships are essential for advancing quantum technologies and our comprehension of the universe's fundamental workings.
5. Material Interaction
Material interaction profoundly influences photon properties. The interaction between photons and matter is a central concept in photondelta, as quantifiable changes in photons often arise directly from encounters with specific materials. A material's composition, structure, and properties directly affect how photons are absorbed, scattered, reflected, or transmitted. These interactions, measurable as photondelta, provide valuable insights into the material's internal structure and dynamics. For example, in spectroscopy, the unique absorption and emission spectra of materials stem from specific energy level transitions that influence the energy of photons, directly correlating with photondelta.
The practical significance of understanding material-photon interactions is substantial. In material science, analyzing photondelta during material interaction offers a means to identify and characterize materials, including determining their composition, structure, and bonding. Such information is critical in developing new materials with specific optical properties for a wide range of applications, from high-performance optical fibers to advanced solar cells. In medical imaging, analyzing photondelta in biological tissue provides insights into the tissue's composition and structure, enabling diagnosis and treatment planning. Similarly, in environmental monitoring, analyzing light-matter interactions enables the characterization of pollutants and atmospheric conditions, revealing valuable information about environmental health and quality.
In summary, material interaction is an intrinsic component of photondelta. The interaction of photons with matter leads to quantifiable changes, forming the basis for characterizing materials and comprehending their properties. The ability to analyze these interactions and measure photondelta enables numerous advancements in various technological fields and scientific domains, ultimately contributing to a deeper understanding of the complex relationship between light and matter. However, challenges remain in precisely measuring photondelta under diverse and complex material interaction scenarios. Future research should address these complexities to optimize applications in areas such as high-precision materials science and medical imaging.
6. Imaging Enhancement
Imaging enhancement relies on manipulating light-matter interactions, a domain deeply intertwined with photondelta. Precisely quantifying changes in photons (photondelta) empowers sophisticated imaging techniques, enabling advancements in resolution, sensitivity, and contrast. Enhanced visualization yields critical information in diverse fields, from medical diagnostics to materials science research.
- Improved Resolution
Enhanced resolution in imaging translates to sharper images, revealing finer details. This improvement often stems from techniques leveraging photondelta, such as using specific wavelengths of light that interact uniquely with the target subject. For instance, in microscopy, manipulating the energy of photons (photondelta) allows for finer visualization of cellular structures. Similarly, in astronomical observations, precise measurement of subtle variations in photon properties (photondelta) enables the differentiation of faint celestial objects.
- Increased Sensitivity
Enhanced sensitivity in imaging systems allows for the detection of weaker signals. This improvement frequently relies on advancements in photon detection technology, which directly measures minute changes in light effectively quantifying photondelta. High-sensitivity imaging enables the identification of subtle variations in materials, such as those employed in medical imaging, where minute differences in tissue composition can reveal pathological conditions. Similar applications exist in environmental monitoring, enabling detection of contaminants at significantly lower concentrations.
- Enhanced Contrast
Improved contrast in imaging techniques separates subtle differences in the target sample, enabling clearer visualization. This often involves manipulating photondelta to highlight variations in the target's properties. For example, contrasting different tissue types in medical imaging relies on precisely controlling the energy and polarization of light (photondelta), enabling discrimination based on the unique interaction of the photons with the various tissues. Similarly, in material science, differentiating materials based on their unique optical responses through quantifiable changes in photon properties (photondelta) enhances contrast.
- Advanced Imaging Modalities
Sophisticated imaging modalities are frequently developed and refined using photondelta. These approaches employ advanced techniques in manipulating photons, including controlling their energy, momentum, or polarization (all components of photondelta) to generate a signal uniquely suited to the imaging needs of specific applications. This approach leads to significant advancements in medical imaging, such as the development of new imaging modalities that offer non-invasive detection of diseases and conditions with enhanced precision. These advancements are linked to a deeper comprehension of photon behavior, which is fundamentally tied to the concept of photondelta.
In essence, imaging enhancement is intrinsically linked to manipulating light-matter interactions, which are directly quantifiable through photondelta. The refined measurement of these interactions enables sharper, more sensitive, and higher-contrast images, leading to significant advances in medical diagnosis, materials characterization, and numerous other applications. The ongoing exploration and application of these concepts within photondelta will likely continue to drive innovation in imaging technologies.
7. Quantum Computing
Quantum computing leverages quantum phenomena to perform calculations, contrasting with classical computing's reliance on bits. Photons, fundamental particles of light, play a pivotal role in this emerging field. Photondelta, the quantifiable change in photon properties, becomes crucial in controlling and manipulating photons within quantum computing architectures. Precise control over photon energy, momentum, and polarization, all measurable aspects of photondelta, is essential for manipulating qubits, the fundamental units of quantum information. Quantum computing's potential for tackling complex problems relies heavily on the ability to precisely control these changes. For instance, photonic quantum gates, employed in some quantum computing architectures, rely on controlled modifications in photon stateseffectively, on photondeltato perform quantum logic operations.
Practical applications of quantum computing, enabled by manipulating photondelta, are potentially vast. Secure communication protocols based on quantum entanglement, where correlations in photon properties dictate the security of transmission, are a prime example. Moreover, algorithms leveraging quantum computing principles, and facilitated by precise control over photondelta, could revolutionize diverse fields. Drug discovery, materials science, and financial modeling could benefit from drastically accelerated simulations that leverage quantum principles and precise measurements of photon properties. These applications underscore the importance of photondelta in translating the theoretical potential of quantum computing into tangible advancements.
In summary, photondelta is intrinsically tied to quantum computing's potential. Precise measurement and control of photon properties (photondelta) are fundamental for manipulating qubits and performing quantum computations. While challenges remain in scaling quantum computing architectures and developing robust measurement techniques for photondelta, the strong link between these concepts points to a future where the ability to precisely control light properties empowers powerful new computing technologies. The ongoing development of sophisticated quantum technologies ultimately hinges on further advancements in our ability to control and exploit photondelta.
8. Light-matter interaction
Light-matter interaction is fundamental to understanding photondelta. Quantifiable changes in photon properties (photondelta) are often a direct consequence of these interactions. Analyzing how light interacts with matter yields crucial insights into the structure, composition, and dynamics of materials, including processes that lead to changes in photons' properties. This exploration examines key facets of light-matter interaction and their connection to photondelta.
- Absorption
Absorption occurs when photons transfer their energy to a material, causing an excitation of the material's atoms or molecules. This energy transfer corresponds directly to a decrease in the photon's energy, a measurable change that is a component of photondelta. For instance, a material's color arises from selective absorption of specific wavelengths of light, with different wavelengths interacting differently with different materials. This absorption process is quantifiable, providing a method to identify and characterize materials.
- Scattering
Scattering involves the redirection of photons by the material's constituent particles. Changes in a photon's direction, energy, or polarization (all facets of photondelta) result from this interaction. Rayleigh scattering, where shorter wavelengths are scattered more strongly, causes the sky to appear blue. Compton scattering exemplifies how photon energy changes during interactions with electrons. These scattering processes reveal details about the material's structure and composition.
- Emission
Emission occurs when a material releases photons, typically after absorbing energy. The energy of the emitted photons corresponds to the energy difference between the material's excited and ground states. Fluorescent materials emit light at specific wavelengths after absorbing higher-energy radiation. This emission process, measurable in terms of photondelta, is critical in various applications, from spectroscopy to light-emitting diodes (LEDs).
- Reflection and Transmission
Reflection occurs when photons bounce off a material's surface, while transmission involves photons passing through a material. Variations in the reflected or transmitted light (related to photondelta) reflect details about the material's properties, such as its refractive index. Different materials reflect and transmit light differently, and the degree of reflection or transmission is a measure of photondelta in these interactions. For instance, the different colors of a prism result from varied reflection and transmission properties of its material.
In summary, light-matter interaction is central to understanding photondelta. The various ways light interacts with materials absorption, scattering, emission, and reflection/transmission all lead to measurable changes in photon properties. These changes, quantified as photondelta, offer valuable insights into the structure, composition, and behavior of materials. Precise measurement of these interactions facilitates applications in diverse fields, from materials science to medical imaging, leveraging the knowledge gained from how light interacts with matter.
Frequently Asked Questions about Photondelta
This section addresses common inquiries regarding photondelta, a term referring to the quantifiable change in photon properties. These questions explore various aspects of photondelta, including its significance and applications in scientific research and technological advancements.
Question 1: What exactly is photondelta?
Photondelta represents the measurable alteration in a photon's properties, such as energy, momentum, or polarization. This quantification provides a precise means to understand light-matter interactions at a fundamental level. Any change in these properties, when measured, constitutes a photondelta.
Question 2: Why is quantifying photondelta important?
Precise measurement of photondelta is crucial for advancing numerous fields. In material science, it helps identify and characterize materials. In medical imaging, it enhances image resolution and sensitivity. In quantum computing, precise control over photondelta enables manipulation of qubits. In essence, quantifying these changes offers insights into fundamental physics and fosters technological advancement.
Question 3: How is photondelta measured?
Techniques for measuring photondelta vary depending on the specific property being quantified. These techniques often involve advanced detectors and specialized optical systems. Spectroscopic methods, for example, are used to measure energy shifts, while polarization analysis measures changes in the orientation of the electric field vector. Further, sophisticated interferometric setups can measure momentum changes in photons.
Question 4: What are the practical applications of photondelta measurements?
Applications span various disciplines. In materials science, precise measurements of photondelta during material interaction provide detailed information about atomic and molecular structure. In medical imaging, quantifying photondelta enhances diagnostics by producing clearer and more sensitive images of biological tissue. Further, photondelta is essential in developing new quantum technologies, like quantum communication and quantum computing.
Question 5: What are the challenges associated with photondelta measurement?
Precise measurements of photondelta can be challenging, particularly in complex environments. Factors such as noise, signal interference, and the need for extremely sensitive detectors can affect accuracy. Developing highly sensitive and robust measurement technologies remains a key focus of ongoing research and development.
In conclusion, photondelta represents a critical aspect of light-matter interaction. Its precise measurement has significant implications across various scientific and technological disciplines. Continued advancements in measurement techniques will likely lead to further breakthroughs in our understanding of the universe and the development of novel technologies.
The subsequent section delves into the specific technologies enabling these measurements.
Conclusion
This exploration of photondelta has illuminated the multifaceted nature of quantifiable changes in photon properties. Key findings highlight the significance of photondelta in diverse domains. The precise measurement of energy shifts reveals crucial information about material interactions, crucial in material science and spectroscopy. Momentum changes are vital in understanding light-matter scattering and optical device design. Polarization alterations are fundamental to optical communication and complex imaging techniques. The role of photondelta in quantum entanglement underscores the potential for novel quantum technologies. Moreover, analysis of photondelta during material interaction provides a powerful tool for characterizing materials, significantly impacting material science and medical imaging. The analysis of photondelta in these diverse scenarios reinforces its importance across numerous fields, showcasing the potential for advancements in both fundamental physics and practical applications.
Further research into photondelta measurement techniques and their application within intricate environments remains essential. Developing more sophisticated instruments to precisely quantify photondelta under diverse conditions, particularly in complex material interactions, is crucial for furthering advancements in various scientific and technological fields. The continued pursuit of these advancements promises to unlock a deeper understanding of the universe, paving the way for innovative solutions in numerous sectors, from medicine to materials science and beyond. The study and application of photondelta represent a frontier of investigation, and further research in this area is poised to reveal profound insights and drive transformative technological progress.
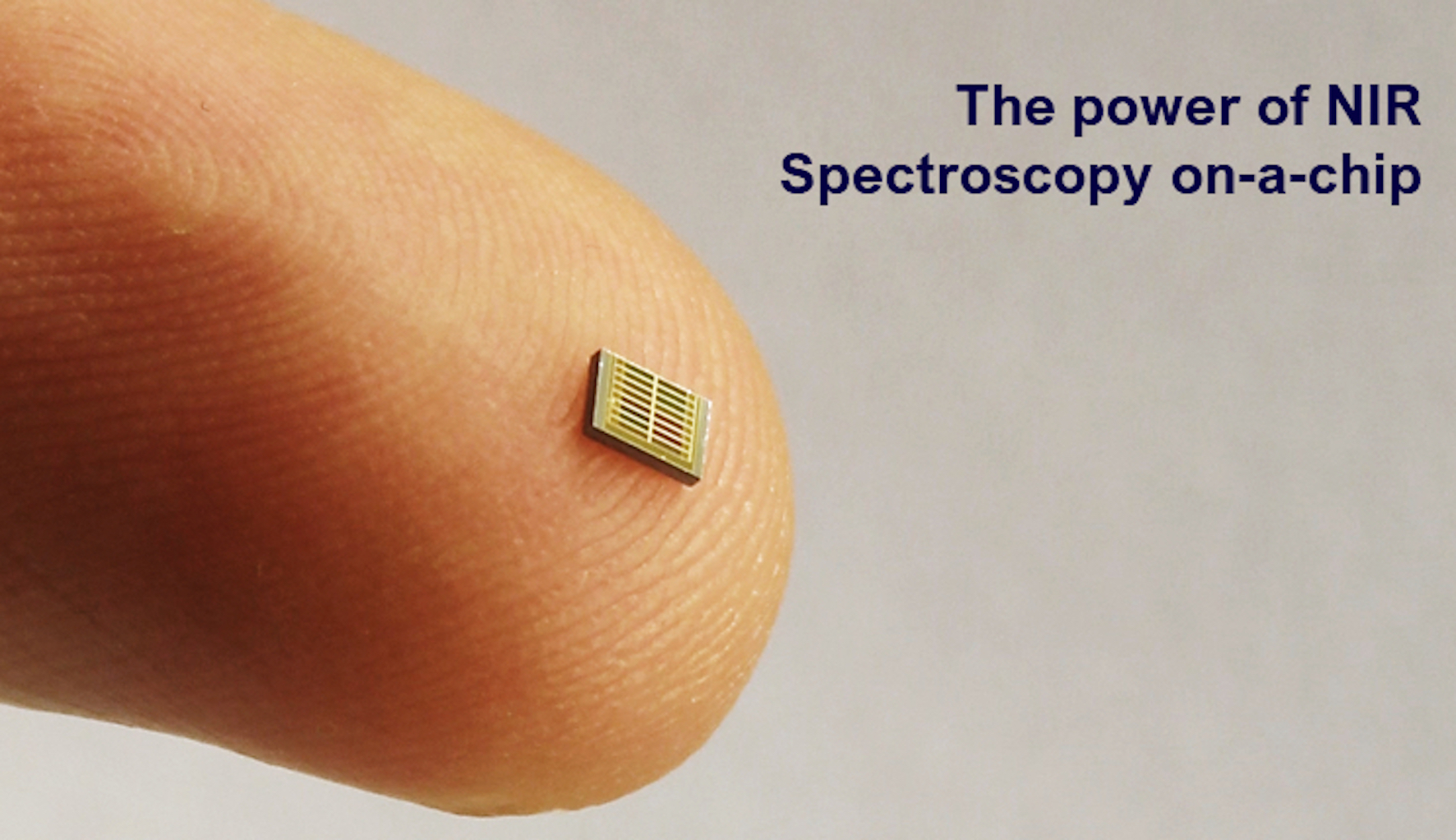
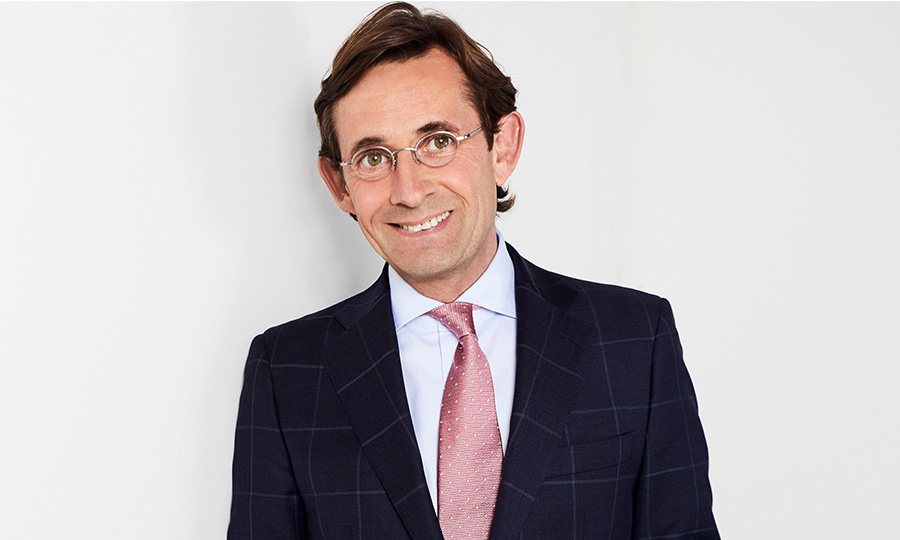

Detail Author:
- Name : Dr. Yoshiko Wiza
- Username : graham.myles
- Email : sanford.palma@sauer.com
- Birthdate : 1989-08-30
- Address : 8082 Rosina Wall Suite 829 Kemmerberg, AL 09785
- Phone : +13603628760
- Company : Nader-Howe
- Job : Paste-Up Worker
- Bio : In sit id ut consequatur aut deserunt porro. Aut molestiae aut ab est. Tempore molestiae necessitatibus tempore quos omnis.
Socials
tiktok:
- url : https://tiktok.com/@raul.kiehn
- username : raul.kiehn
- bio : Recusandae sint commodi dolore dolore atque.
- followers : 381
- following : 1731
instagram:
- url : https://instagram.com/raul_kiehn
- username : raul_kiehn
- bio : Qui magni velit consequatur nemo. Id dignissimos rerum velit nihil soluta.
- followers : 6922
- following : 537
twitter:
- url : https://twitter.com/rkiehn
- username : rkiehn
- bio : Quas autem et qui vel id quae. Est modi tenetur sed consectetur. Iure ut atque quis omnis fugiat.
- followers : 6643
- following : 30
linkedin:
- url : https://linkedin.com/in/raul_dev
- username : raul_dev
- bio : Voluptate exercitationem nihil velit odit.
- followers : 730
- following : 442
facebook:
- url : https://facebook.com/rkiehn
- username : rkiehn
- bio : Quam error voluptatem quia cupiditate et molestiae dolorum.
- followers : 6290
- following : 1532