What is the advanced material enabling structural innovations? A revolutionary building block promises strength and sustainability.
This material, a composite construction component, combines high compressive strength with lightweight properties. Utilizing advanced materials science, the component achieves exceptional performance characteristics through unique structural design. Examples include applications in high-rise structures and specialized industrial settings, where weight reduction and enhanced stability are paramount. Its development signifies a significant advancement in construction techniques, potentially reshaping architectural possibilities.
The benefits of this material are multifaceted. Reduced material usage translates to lower environmental impact during production and transportation. Its enhanced load-bearing capacity allows for the design of more efficient and spacious structures. Its inherent durability reduces long-term maintenance requirements, providing cost savings over the lifespan of the structure. The material has the potential to impact urban development by enabling construction in challenging landscapes or constrained urban spaces.
- Stunning Ebony Chaturbate Models Hot Videos
- Former President Trumps Signature Authentic Examples Analysis
Let's delve deeper into the technical aspects of this innovative structural component. We'll explore the specific design principles behind its exceptional performance and examine various applications.
psi brick
Understanding the key aspects of this advanced building material is crucial for appreciating its potential impact. A thorough analysis reveals core characteristics that influence its application and performance.
- Strength
- Lightweight
- Durability
- Sustainability
- Design flexibility
- Cost-effectiveness
These six aspects define the material's efficacy. High strength and lightweight construction allow for taller, more spacious structures. Durability minimizes long-term maintenance, while sustainability reduces environmental impact through material efficiency. Design flexibility enables innovative building designs, and potential cost savings arise through reduced material usage and maintenance needs. The material's ability to combine these characteristics directly translates to significant improvements in construction efficiency and sustainability, particularly in high-rise construction where weight and cost are critical considerations.
1. Strength
The compressive strength of the material is a defining characteristic. This strength, measured in pounds per square inch (psi), directly dictates the material's load-bearing capacity. A high psi rating allows the material to withstand substantial weight and stress, a critical factor in structural integrity. This crucial property enables its application in load-bearing walls, floors, and other structural elements in high-rise buildings and other demanding environments.
The design of the material itself must account for stress distribution to achieve optimal strength. Sophisticated engineering principles dictate the arrangement of internal components to maximize resilience. Real-world examples illustrate this: In bridge construction, the strength of the material is paramount; similarly, the stability of skyscrapers relies heavily on the compressive strength of the materials used in their construction. This understanding is fundamental in preventing structural failures and ensuring the safety of occupants and the surrounding environment.
In summary, the strength of the material is not just a desirable characteristic, but a fundamental necessity for practical application. Its impact on structural integrity and safety is undeniable. Engineers and architects must rigorously evaluate the compressive strength to ensure suitability for diverse construction projects.
2. Lightweight
The lightweight nature of this material is a crucial component of its design. Reduced weight directly impacts structural efficiency. A lighter material requires less supporting structure, leading to potential cost savings and increased design flexibility. This reduced weight also decreases transportation costs, aligning with sustainability goals. Consequently, the material's application in high-rise construction becomes more feasible, enabling architects to design taller, more open spaces without compromising structural integrity. The reduction in material mass facilitates easier construction and installation, especially in challenging terrain. Furthermore, lighter materials contribute to a reduced carbon footprint during construction and the material's overall lifecycle.
Practical applications of this lightweight property are numerous. In high-rise buildings, the reduced weight of the building materials leads to smaller and more economical foundation designs. This translates into substantial cost savings, particularly in densely populated urban areas. Moreover, lightweight construction facilitates faster and more efficient building processes, potentially accelerating project completion timelines. In bridge construction, lightweight materials enable the construction of longer spans with fewer supporting pillars, enhancing aesthetic appeal and structural stability. The material's reduced weight is critical for deployment in remote or difficult-to-access locations, where transportation limitations significantly impact traditional construction methods.
The material's combination of high strength and low weight stands as a significant advancement in construction technologies. This fusion allows for innovative architectural designs and more sustainable construction practices. Understanding the interconnectedness of lightweight properties and material strength enables engineers and architects to optimize structural designs for specific project requirements. Further research and development in this area could unlock new opportunities for sustainable, efficient, and cost-effective infrastructure development globally. Moreover, these attributes position the material as a valuable tool for responding to pressing societal challenges related to urban development, environmental sustainability, and resource efficiency.
3. Durability
Durability, a critical aspect of any construction material, is intrinsically linked to the performance of this material. Long-term resistance to environmental factors, such as weathering and degradation, directly impacts the material's lifespan and structural integrity. In this context, durability is not merely a desirable feature but a fundamental necessity for the material's sustained effectiveness in diverse applications. The resistance to wear, tear, and environmental stresses is crucial for the structural stability and functionality of constructed elements over extended periods.
The specific mechanisms contributing to the durability of this material are multifaceted and complex. Factors like material composition, manufacturing processes, and design elements interact to create a composite effect, influencing its resilience against degradation. Examples include its resistance to moisture absorption, its ability to resist chemical attack from exposure to various substances, and its resistance to mechanical stresses over time. These attributes are essential for ensuring longevity in demanding environments, from coastal regions exposed to saltwater to industrial settings with chemical exposure. Careful consideration of these factors in the design and construction phases significantly contributes to the material's ability to withstand the rigors of its intended use, ultimately prolonging the useful life of constructed structures. Real-world applications demonstrate how this durability translates into reduced maintenance needs and extended service lives, ultimately affecting construction costs and project timelines.
In conclusion, durability is a key performance indicator for this material. The ability to withstand environmental stresses, mechanical loads, and chemical attacks is fundamental to its long-term effectiveness. Understanding the interplay of material composition, manufacturing procedures, and structural design is crucial for achieving and sustaining high durability. This knowledge allows for informed material selection and promotes the creation of resilient and enduring structures, contributing to a more sustainable construction industry.
4. Sustainability
The connection between sustainability and this advanced building material is profound. Reduced environmental impact is a direct consequence of the material's design and application. Lower material consumption, resulting from enhanced load-bearing capacity, minimizes the extraction of raw materials. Efficient material utilization decreases the environmental burden of transportation and manufacturing, a crucial element in sustainable construction practices. Minimizing waste during construction and throughout the material's lifecycle aligns with the core principles of environmental sustainability. In contrast to traditional construction methods reliant on substantial resource consumption, this material represents a transition towards more environmentally responsible practices.
Practical examples illuminate the significance of this connection. In urban environments, where space is often limited and resource management is paramount, the material's reduced weight allows for taller structures and more efficient land use. This translates to reduced need for land development and minimized urban sprawl, mitigating the negative environmental consequences often associated with urban expansion. Reduced waste generation through optimized material use also contributes to lower landfill volumes and a more circular economy. The material's inherent durability, coupled with its high performance, extends the lifespan of structures, minimizing the need for premature replacements and the associated environmental cost. Sustainable construction practices like this are essential for mitigating the environmental impact of built environments.
Ultimately, the incorporation of sustainability into the design and application of this building material underscores a paradigm shift in construction. The focus on resource efficiency, minimized waste, and extended material lifecycles are crucial components of long-term environmental responsibility. By embracing these principles, the construction industry can contribute to a more sustainable future while meeting the demands of modern infrastructure needs. However, challenges remain, including the scalability of production processes, the accessibility of raw materials, and consumer acceptance of innovative building materials. Overcoming these challenges will further cement the material's role as a cornerstone of sustainable construction practices.
5. Design Flexibility
Design flexibility, a key attribute of this advanced building material, significantly impacts architectural possibilities. The material's unique properties enable innovative structural designs, surpassing the limitations of traditional construction methods. This exploration delves into the specific ways this flexibility manifests and its implications for construction projects.
- Variable Load-Bearing Capacity
The material's adjustable strength allows architects to design structures with varying load distributions. This adaptability enables innovative architectural designs not possible with traditional materials. For instance, more open floor plans with fewer internal load-bearing walls can be realized. The material's capability to handle different structural demands provides increased design freedom. This adaptability is crucial for creating bespoke structures tailored to unique functional requirements or aesthetic preferences.
- Optimized Space Utilization
The material's lightweight nature enables the creation of more spacious and efficient designs. The reduced structural demands allow for greater internal volume, leading to more flexible interior layouts. This is especially significant in high-rise construction, where maximizing usable space is paramount. This aspect of flexibility allows architects to design structures maximizing available space and offering potential for more adaptable interior layouts.
- Diverse Architectural Forms
Design freedom extends to the material's potential for intricate and non-traditional shapes. Complex geometries previously challenging to realize using traditional methods become achievable. This flexibility permits the construction of more aesthetically striking and visually interesting buildings. This adaptability to varied forms offers architects the ability to create designs that are not only structurally sound but also visually captivating, potentially fostering more creative and innovative architecture.
- Adaptability to Site Conditions
The material's inherent versatility accommodates varying site conditions. Its ability to handle varied terrain and environmental pressures allows for designs that respond to local contexts. This adaptability is essential in challenging or constrained urban landscapes where traditional methods might prove restrictive. Specific site constraints can be addressed more readily through this flexibility, offering practical solutions for construction in locations with particular conditions, maximizing project feasibility in challenging environments. This adaptability contributes to the material's broader applicability in various geographic and environmental settings.
In conclusion, the design flexibility inherent in this material is instrumental in realizing innovative and cost-effective architectural designs. The interplay of strength, weight, and adaptability provides architects and engineers with the tools to create more efficient, adaptable, and visually compelling structures. This is critical in pushing the boundaries of modern construction, contributing to more sustainable and innovative architectural solutions. The adaptability of this material to diverse conditions allows for designs responsive to specific project requirements and location specifics, ultimately advancing the potential of design possibilities within structural engineering.
6. Cost-effectiveness
The cost-effectiveness of this advanced building material hinges on its ability to deliver enhanced structural performance at a lower overall project cost. This involves evaluating not only the material's initial price but also its impact on various stages of a construction project, including labor, transportation, and long-term maintenance.
- Reduced Material Requirements
The material's high strength-to-weight ratio necessitates less material to achieve the same structural integrity compared to traditional building blocks. This translates to reduced quantities of raw materials, potentially lowering the material cost component of the project. Less material also translates to reduced transportation and handling costs, which can be significant, especially for large-scale projects.
- Optimized Labor Costs
The material's enhanced structural properties often facilitate faster construction. Reduced material handling and simpler assembly processes result in a potential decrease in labor time and, consequently, labor costs. This efficiency can be particularly pronounced in projects with tight deadlines, contributing to cost savings and project timetabling.
- Minimized Long-Term Maintenance
Enhanced durability, an inherent characteristic of the material, often leads to lower long-term maintenance needs. Structures built with this material demonstrate exceptional resistance to weathering, degradation, and other factors that require regular maintenance in conventional designs. This reduced maintenance frequency and scope translate into substantial savings over the lifespan of the structure.
- Potential for Alternative Design Approaches
The material's unique properties enable architects to explore alternative design approaches. This flexibility often results in lighter structures and less-complex designs, minimizing the use of secondary reinforcement materials or elements. This alternative design approach can translate into significant cost savings in materials and labor.
In conclusion, the cost-effectiveness of this composite building material emerges from its synergistic effect on various project components. By simultaneously enhancing structural performance, reducing material use, and lowering long-term maintenance demands, the material ultimately offers a potentially more economical solution for construction projects compared to traditional building techniques. Factors such as specific project requirements, site conditions, and design specifications, however, significantly influence the precise cost-effective evaluation. This comprehensive analysis allows for a deeper understanding of the material's practical application and potential impact on the economics of construction projects.
Frequently Asked Questions about "Psi Brick"
This section addresses common inquiries regarding the advanced composite construction material, "psi brick," highlighting key aspects and dispelling potential misconceptions.
Question 1: What distinguishes "psi brick" from traditional building materials?
The defining characteristic of "psi brick" lies in its exceptional strength-to-weight ratio. This is achieved through advanced material science and design, enabling taller, more open structures with reduced material usage compared to conventional building blocks. This also allows for optimized load distribution, resulting in more flexible design options. The material's durability and sustainability further differentiate it, minimizing long-term maintenance requirements and promoting resource efficiency.
Question 2: What are the environmental benefits of using "psi brick"?
Reduced material usage minimizes the extraction of raw materials, thereby lessening the environmental impact associated with resource extraction. The material's lightweight nature lowers transportation costs and energy consumption during construction. Furthermore, the enhanced durability translates to a longer lifespan for structures, minimizing the frequency of replacements and associated environmental costs.
Question 3: How does the strength of "psi brick" compare to traditional materials?
Studies demonstrate "psi brick" often surpasses traditional masonry in terms of compressive strength. The material's advanced composite structure allows it to withstand significant loads while maintaining a relatively low weight. This translates to the potential for taller structures with reduced foundation requirements.
Question 4: What are the potential applications for "psi brick" in construction?
The material's strength-to-weight ratio, durability, and design flexibility make it suitable for diverse construction applications. This includes high-rise buildings, bridges, and specialized industrial structures. The material's adaptability to various site conditions enhances its practicality in diverse geographic locations.
Question 5: What are the current limitations of "psi brick" technology?
While promising, the scalability of production processes and the availability of raw materials may present challenges in widespread adoption. Further research and development might be required to fully address potential limitations and optimize the material's application across various construction contexts.
Understanding these frequently asked questions provides a comprehensive overview of the potential and challenges surrounding "psi brick" technology, highlighting its potential in shaping the future of construction.
This concludes the FAQ section. The following section will delve into the technical specifications of "psi brick".
Conclusion
The exploration of "psi brick" reveals a material with significant potential for transforming construction practices. Key attributes, including exceptional strength-to-weight ratios, enhanced durability, and design flexibility, position this composite material as a viable alternative to traditional building blocks. The material's capacity for reduced material usage and optimized structural designs offers substantial potential for sustainable construction, aligning with evolving environmental priorities. Cost-effectiveness, stemming from reduced material requirements, minimized labor costs, and potentially lower long-term maintenance, further underscores the economic advantages of this innovation. However, scalability of production and accessibility of raw materials remain critical considerations for widespread adoption. The material's ability to respond to variable site conditions, enabling adaptable designs in diverse architectural contexts, underscores its potential for broader applications.
Further research and development efforts are essential to fully realize the potential of "psi brick." This includes refining manufacturing processes for scalability, optimizing the material's composition for diverse applications, and conducting comprehensive long-term performance evaluations. Ultimately, embracing innovation in materials science, like "psi brick," presents a critical pathway towards more sustainable, resilient, and cost-effective infrastructure development. The future of construction hinges on the integration of such advanced materials, offering a responsible and impactful approach to modern development.


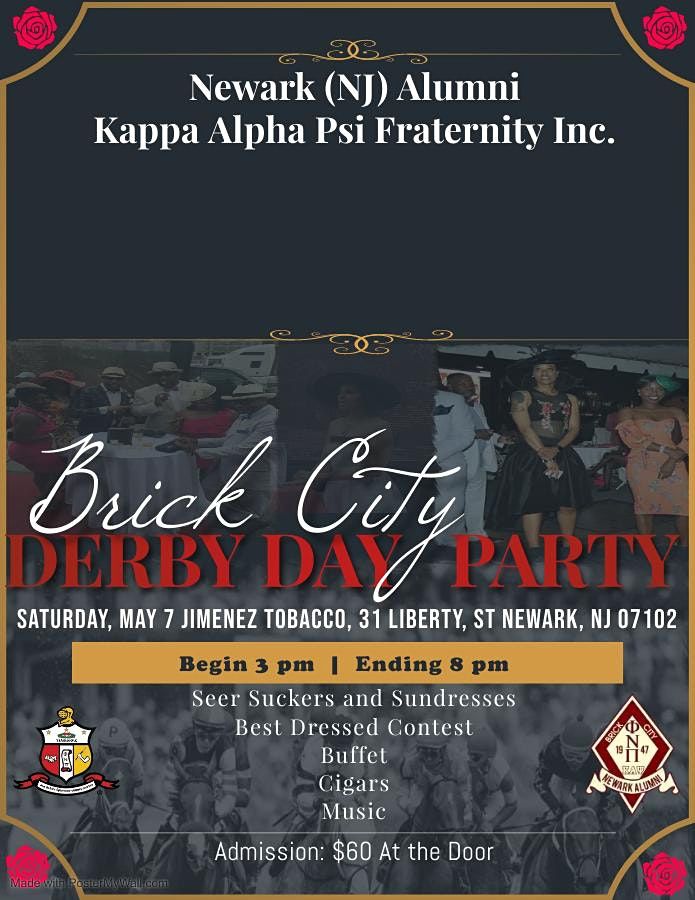
Detail Author:
- Name : Dr. Erna Hartmann I
- Username : rsmith
- Email : brendan21@hotmail.com
- Birthdate : 1985-04-13
- Address : 5223 Ankunding Camp Suite 431 East Iciemouth, OK 17773-2469
- Phone : (952) 724-0413
- Company : Terry-Sawayn
- Job : Drilling and Boring Machine Tool Setter
- Bio : Et voluptas labore beatae quod cum ea odio. Autem aut minus nesciunt quo veniam autem nostrum. Nemo dolorem quis dignissimos recusandae omnis est. Necessitatibus velit et quasi neque tempore.
Socials
facebook:
- url : https://facebook.com/verdiesanford
- username : verdiesanford
- bio : Pariatur aut a non sapiente omnis eos.
- followers : 5667
- following : 14
linkedin:
- url : https://linkedin.com/in/verdie_sanford
- username : verdie_sanford
- bio : Voluptatem dolorem maiores eum enim sint.
- followers : 5807
- following : 1787
twitter:
- url : https://twitter.com/verdie_id
- username : verdie_id
- bio : Asperiores nemo aliquid ea eligendi. Qui deleniti facere eligendi modi natus autem iusto ipsum. Sint quae reprehenderit rerum dolorum fugiat ut.
- followers : 6979
- following : 791
tiktok:
- url : https://tiktok.com/@verdiesanford
- username : verdiesanford
- bio : Praesentium non nihil et modi maiores nulla.
- followers : 6741
- following : 2128
instagram:
- url : https://instagram.com/sanfordv
- username : sanfordv
- bio : Sapiente quo suscipit rerum molestiae deserunt dolore voluptatem. Quos quia et sunt pariatur.
- followers : 459
- following : 416